29th January 2023, Dr Chee L Khoo
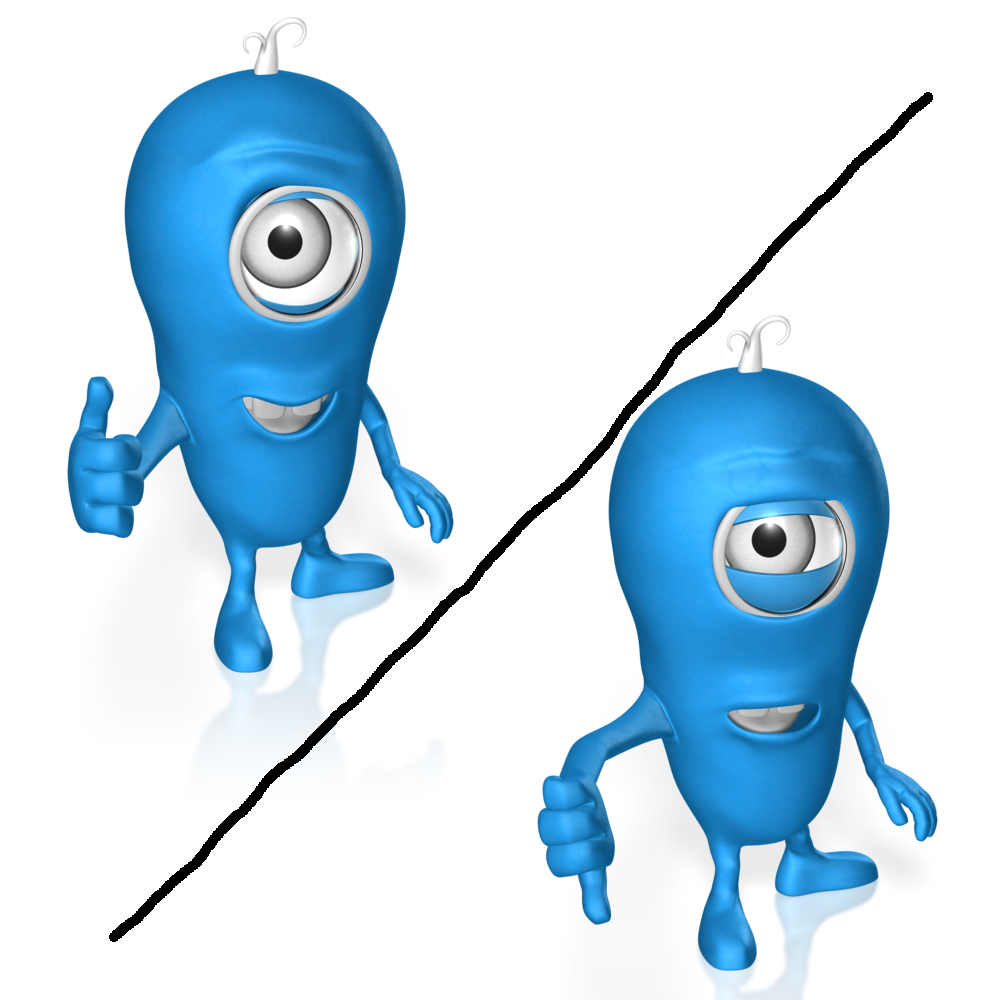
We have always regarded HDL-Cholesterol (HDL-C) as the good guys. In the 1970s, the Framingham Heart Study showed that HDL-C was negatively associated with the development of cardiovascular disease (CVD) (1). Thus, we have regarded HDL as anti-atherogenic lipoprotein and expected HDL-C-raising therapy to reduce CVD events. However, there have been recent reports of the associations of high HDL-C and osteoporosis and fractures in healthy older adults. There have also been recent reports on the association between very high HDL-C and the paradoxically increased mortality in patients with coronary artery disease. Suddenly, the status of the “good” cholesterol is thrown into question.
What does HDL-C do?
Researchers used to focus on the size and density of HDL-C and the proteins and phospholipids it contains, classifying them by density gradient ultracentrifugation, two-dimensional gel electrophoresis, nuclear magnetic resonance spectroscopy, or ion mobility [2]. Recent technologies, including proteomics and lipidomics, have uncovered the heterogeneity and complexities of HDL-C.
HDL-C transports excess cholesterol from foamy macrophages and smooth muscle cells and peripheral tissues to the liver. Apolipoprotein A-I (ApoA-I), a major apolipoprotein of HDL-C, is mainly secreted from the liver and small intestine. The secreted apoA-I gains phospholipids and cholesterol via ATP binding cassette transporter A1 (ABCA1) from peripheral tissues to form discoidal HDL (pre-HDL) and further effluxed cellular cholesterol via ATP binding cassette transporter G1 (ABCG1), and SR-BI, which leads to the formation of mature HDL particles by lecithin cholesterol acyltransferase (LCAT).
HDL-C is either stored as cholesterol esters or excreted in the bile. The majority of cholesterol ester (HDL-CE) is transferred from HDL to VLDL and LDL by cholesteryl ester transfer protein (CETP) and is taken up by LDL receptor (LDLR) in the liver. This reverse cholesterol transport system is believed to have a major anti-atherosclerotic function.
The other functions of HDL-C
Atherosclerosis progression is associated with inflammation and HDL-C can exert anti-inflammatory actions. Monocyte recruitment is known to be a first step in atherosclerosis formation. Cockerill et al. reported that HDL-C exerted anti-inflammatory effects via reducing cytokine-induced adhesion molecules, such as VCAM1 (vascular cell adhesion molecule-1) [3]. Murphy et al. focused on anti-inflammatory effects on immune cells and reported that HDL-C and ApoA-1 attenuated neutrophil activation in vitro and in vivo [4]. From another point of view, ABCA1- and ABCG1-mediated cholesterol efflux due to HDL-C was reported to be modulated by the immune cell response.
HDL-C can remove lipid hydroperoxide (LOOH) and oxidised phospholipids (oxPL) from atherogenic oxidised LDL (oxLDL). Davidson et al. focused on the association of HDL-C composition with anti-oxidative functions and found that apoD, apoF, apoJ, apoL1, and apo M were associated with LDL oxidation [5].
HDL regulates endothelial nitrous oxide synthase (eNOS), thereby modulating endothelial homeostasis. Endothelial dysfunction is mainly involved in early atherosclerosis progression by disrupting homeostasis of nitric oxide production [6].
Observational studies has established that HDL-C is inversely associated with both cardiovascular disease and mortality across a wide range of concentrations (7-9). You would have thought that increasing HDL-C would be beneficial. However, raising HDL-C pharmacologically has not proven beneficial in randomised clinical trials and has even paradoxically been associated with increased mortality in one study (10,11). Furthermore, in patients with certain genetic variants of the CETP gene which is associated with elevated levels of HDL-C, the risk of cardiovascular disease is paradoxically elevated. In other patients, a loss of function mutation in a major HDL receptor, Scavenger Receptor B1 (SCARB1), which lead to high concentrations of HDL cholesterol, was associated with high risk of coronary heart disease (12).
The above “other functions” of HDL-C has been shown to be adversely affected in various disease states (e.g. diabetes, chronic kidney disease, autoimmune conditions, cancers) independent from the effect on HDL-C count. Perhaps, the studies have not allowed for less obvious confounding factors.
The U-shaped curve
There is evidence suggesting that the association between HDL cholesterol on a continuous scale and all-cause mortality is U-shaped for men and women, as both low and high concentrations were associated with high all-cause mortality. In fact, we have known about this U-shaped curve for some time.
Madsen et al presented data from two prospective studies from Denmark in 2017 (13). A total of 52 268 men and 64 240 women were included from the two prospective population-based studies, the Copenhagen City Heart Study and the Copenhagen General Population Study. Overall, over 745 452 person-years of follow-up, the association between HDL-C concentrations and all-cause mortality was U-shaped for both men and women, with both extreme high and low concentrations being associated with high all-cause mortality risk.
The concentration of HDL-C associated with the lowest all-cause mortality was 1.9 mmol/L in men and 2.4 mmol/L in women. When you compare this group with the lowest risk, men who had HDL-C of 2.5–2.99 mmol/L, adjusted hazard ratios for all-cause mortality were 1.36. When you compare the same lowest risk with HDL cholesterol ≥3.0 mmol, the adjusted hazard ratio for all cause mortality were 2.06. For women, the corresponding hazard ratios were 1.10 for HDL cholesterol of 3.0–3.49 mmol/L and 1.68 for HDL-C ≥3.5 mmol/L.
When they break down the cause specific mortality, there were some surprises. For cardiovascular disease endpoints ischaemic heart disease and myocardial infarction, a high risk was observed for low concentrations of HDK-C. There was a plateau around HDL-C of 1.5 mmol/L and 2.0 mmol/L for men and women, respectively, with no further decrease in risk with concentrations of HDL-C higher than that. No significant increase in these risks were observed with extreme high concentrations of HDL-C. For ischaemic stroke similar patterns of association were observed.
The authors postulated that perhaps, patients with extremely high HDL-C may have extreme high HDL-C due to a genetic variant. Genetic variants may have detrimental effects causing high risk of disease and death, which is the case for certain mutations in CETP, ABCA1, LIPC, and SCARB1, which are associated with both high risk of coronary heart disease and high concentrations of HDL-C. Perhaps genetic variants, which increases risk in ways we do not understand and also increases HDL, suggesting that the physiology of HDL is complex and perhaps not well understood.
The findings from the Danish study are supported by pooled analysis from the Evidence for Cardiovascular Prevention from Observational Cohorts in Japan (EPOCH-Japan) which included nine cohort studies in Japan [14].
In a separate cohort of patients, 14 478 participants with CAD enrolled in the UK Biobank (UKB) and 5467 participants in the Emory Cardiovascular Biobank (EmCAB), individuals in the UKB with HDL-C levels > 2.0 mmol/L had a 96% higher risk of all-cause mortality and a 71% higher risk of cardiovascular mortality after adjustment for covariates, compared with those with HDL-C levels in the range of 1.0 – 1.5 mmol/L (15).
It would seem that focussing on HDL-C concentrations alone may not be sufficient in assessing whether HDL-C is a good guy or a bad guy. HDL-C not only transports cholesterol away from macrophages, smooth muscles and endothelium. It has other functions which may be affected in different disease states and may adversely affect cardiovascular and all-cause mortality. Numerous clinical and basic studies have shown that HDL functions are impaired in various diseases and environments. Furthermore, HDL has become a potential therapeutic target for cardiovascular disease and cancer. In future research, a comprehensive understanding of HDL functionality will be essential for unveiling new aspects of HDL.
References:
1. Kannel, W.B.; Castelli, W.P.; Gordon, T.; McNamara, P.M. Serum cholesterol,
lipoproteins, and the risk of coronary heart disease. The Framingham study.
Ann. Intern. Med. 1971, 74, 1–12.
2. Rosenson, R.S.; Brewer, H.B., Jr.; Chapman, M.J.; Fazio, S.; Hussain, M.M.; Kontush, A.; Krauss, R.M.; Otvos, J.D.; Remaley, A.T.; Schaefer, E.J. HDL measures, particle heterogeneity, proposed nomenclature, and relation to atherosclerotic cardiovascular events. Clin. Chem. 2011, 57, 392–410.
3. Cockerill, G.W.; Rye, K.A.; Gamble, J.R.; Vadas, M.A.; Barter, P.J. High-density lipoproteins inhibit cytokine-induced expression of endothelial cell adhesion molecules. Arterioscler. Thromb. Vasc. Biol. 1995, 15, 1987–1994.
4. Murphy, A.J.; Woollard, K.J.; Suhartoyo, A.; Stirzaker, R.A.; Shaw, J.; Sviridov, D.; Chin-Dusting, J.P. Neutrophil activation is attenuated by high-density lipoprotein and apolipoprotein A-I in in vitro and in vivo models of inflammation. Arterioscler. Thromb. Vasc. Biol. 2011, 31, 1333–1341.
5. Davidson, W.S.; Silva, R.A.; Chantepie, S.; Lagor, W.R.; Chapman, M.J.; Kontush, A. Proteomic analysis of defined HDL subpopulations reveals particle-specific protein clusters: Relevance to antioxidative function. Arterioscler. Thromb. Vasc. Biol. 2009, 29, 870–876.
6. Davignon, J.; Ganz, P. Role of endothelial dysfunction in atherosclerosis. Circulation 2004, 109, Iii27–Iii32.
7. Di Angelantonio E , Sarwar N , Perry P , Kaptoge S , Ray KK , Thompson A , Wood AM , Lewington S , Sattar N , Packard CJ , Collins R , Thompson SG , Danesh J. Major lipids, apolipoproteins, and risk of vascular disease. Jama 2009;302:1993–2000
8. Wilson PW , Abbott RD , Castelli WP. High density lipoprotein cholesterol and mortality. The Framingham Heart Study. Arteriosclerosis 1988;8:737–741
9. Pekkanen J , Linn S , Heiss G , Suchindran CM , Leon A , Rifkind BM , Tyroler HA. Ten-year mortality from cardiovascular disease in relation to cholesterol level among men with and without preexisting cardiovascular disease. N Engl J Med 1990;322:1700–1707
10. Keene D , Price C , Shun-Shin MJ , Francis DP. Effect on cardiovascular risk of high density lipoprotein targeted drug treatments niacin, fibrates, and CETP inhibitors: meta-analysis of randomised controlled trials including 117,411 patients. Bmj 2014;349:g4379.
11. Barter PJ , Caulfield M , Eriksson M , Grundy SM , Kastelein JJ , Komajda M , Lopez-Sendon J , Mosca L , Tardif JC , Waters DD , Shear CL , Revkin JH , Buhr KA , Fisher MR , Tall AR , Brewer B , Investigators I. Effects of torcetrapib in patients at high risk for coronary events. N Engl J Med 2007;357:2109–2122
12. Zanoni P , Khetarpal SA , Larach DB , et al. Consortium CHDE, Consortium CAE, Global Lipids Genetics C. Rare variant in scavenger receptor BI raises HDL cholesterol and increases risk of coronary heart disease. Science 2016;351:1166–1171.
13. Madsen, C.M.; Varbo, A.; Nordestgaard, B.G. Extreme high high-density lipoprotein cholesterol is paradoxically associated with high mortality in men and women: Two prospective cohort studies. Eur. Heart J. 2017, 38, 2478–2486.
14. Hirata, A.; Sugiyama, D.;Watanabe, M.; Tamakoshi, A.; Iso, H.; Kotani, K.; Kiyama, M.; Yamada, M.; Ishikawa, S.; Murakami, Y.; et al. Association of extremely high levels of high-density lipoprotein cholesterol with cardiovascular mortality in a pooled analysis of 9 cohort studies including 43,407 individuals: The EPOCH-JAPAN study. J. Clin. Lipidol. 2018, 12, 674–684.e675.
15. Liu C, Dhindsa D, Almuwaqqat Z, et al. Association Between High-Density Lipoprotein Cholesterol Levels and Adverse Cardiovascular Outcomes in High-risk Populations. JAMA Cardiol. 2022;7(7):672–680. doi:10.1001/jamacardio.2022.0912