13th July 2024, A/Prof Chee L Khoo
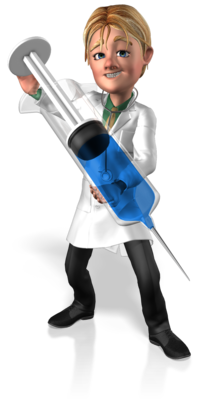
Iron deficiency can occur in a number of scenarios. It can arise from blood loss (gastrointestinal, gynaecological), inadequate dietary sources or limited absorption in inflammatory conditions (e.g CKD, heart failure or diabetes). Oral iron supplements not only can have annoying GI side effects but absorption is poor under the best of conditions. Further, absorption doesn’t work that well in inflammatory conditions because of elevated hepcidine. Often, we have to resort to intravenous iron infusions both for efficacy reasons and speed to response. We don’t think about how the iron actually gets utilised by the tissues. A recent prospective study into how different organs handled the infused iron differently and there were a few surprises which will make us think about the “safety” of iron infusion.
Fate of iron when infused
Intravenous iron therapies contain iron–carbohydrate complexes, designed to ensure iron becomes bioavailable via the intermediary of spleen and liver reticuloendothelial macrophages. After intravenous iron infusion, ferric carboxymaltose (FCM) can end up in three places:
- Taken up by reticuloendothelial macrophages of the spleen and liver. FCM is metabolized and iron liberated for export into the circulation by the iron exporter ferroportin (FPN). Upon export, this iron is loaded onto its plasma transferrin (Tf). This transferrin-bound iron is then consumed, primarily by the bone marrow for the production of red blood cells. Because plasma ferritin derives primarily from macrophages, uptake of iron–carbohydrate complexes by reticuloendothelial macrophages is closely followed by a rise in plasma ferritin. This is called the canonical pathway.
- Iron is also stored in the liver to be available in times of increased iron demand
- FCM also releases iron directly into the circulation to be taken up by non-transferrin-bound-iron (NTBI). These are then taken up by myocytes via the abundant L-type and T-type calcium channels (L/TTCC) and divalent metal transporter. The iron that enters the cardiac myocytes in this manner joins the intracellular labile iron pool, which is the pool of reactive iron responsible for generating oxidative stress.
The surface expression of transferrin receptor (TfR) is coupled to cellular iron need, because its levels are controlled by the iron homeostatic proteins iron regulatory proteins. NTBI transporters are not regulated by IRPs. They continue to take up iron even when there is excess cellular labile iron.
Potentially, repeated iron infusion may lead to iron overload within myocytes resulting in iron-overload cardiomyopathy while the plasma ferritin remains low. Tissue iron uptake can be measured by changes in MRI signals (MR Relaxation)
The Study of Tissue Iron Uptake in iron-deficient patients following IV iron therapy (STUDY) is an investigator-initiated, prospective, observational study conducted at one UK site, sponsored by the University of Oxford aiming to investigate myocardial uptake and handling of iron following IV iron therapy with the most widely used formulation FCM (1).
12 patients with iron deficiency (Ferritin < 100 µg/L and /or Saturation < 20%) were recruited to receive 1000mg of FCM by slow IV infusion. The primary outcome was changes from baseline in multi-organ MR relaxation times T1, T2, and T2* for each participant. participants were scanned immediately prior to infusion (0 h), then 3 h, 14 days, and 42 days post-infusion The secondary outcome was changes from baseline in plasma iron indices: iron, ferritin, Tsat, NTBI, and serum levels of lipid peroxidation marker malondialdehyde (MDA).
Results
After a single standard dose of FCM myocardial iron rose rapidly and maximally within 3 h, and this rise is sustained for at least 42 days post-infusion. After a single dose of FCM, spleen iron begins to rise within 3 h, but rises maximally at 14 days, and is declining again at 42 days. In the liver, iron rose within 3 hours but returned to normal by 14 days.
With plasma iron, transferrin and ferritin, they all went up significantly within 3 hours and reached maximal levels by 14 days. Between 14 days and 42 days, the levels declined.
When they repeated a similar study in mice, they were able to measure labile iron pool in the myocardium. In FCM-treated iron-deficient mice, myocardial LIP was raised both 1 h after infusion (P = .028 vs. saline) and 42 days after infusion.
This study confirmed that the rise in myocardial iron is the result of direct uptake of FCM from the circulation via NTBI rather than via the canonical pathway involving the reticuloendothelial macrophage. The accumulation of iron within the myocardium appears to still be present at the 42 day mark. This retention of myocardial iron is in contrast to the iron in the spleen and liver. The iron in these organs begin to decline by day 42.
The findings of the current study have important clinical implications. While the increase in myocardial iron following a single dose of FCM is not of the magnitude known to cause cardiomyopathy (2) the lack of iron clearance from the myocardium over the period of 42 days highlights the potential for cumulative build-up with repeated doses. What happens to the accumulated iron in the myocardium after repeated iron infusion? This raises pertinent questions about the potential for cardiac complications in those receiving long-term IV iron therapy.
A few settings comes to mind. Women receiving multiple iron infusion for heavy uterine bleeding, patients with chronic kidney disease and patients with heart failure receiving repeated iron infusions. In patients on dialysis, high IV iron dosing regimens of >300 mg/month have been associated with higher mortality (3). On the other hand, trials of long-term IV iron therapy in patients with HF (where up to nine doses were administered) have not reported an increased risk of adverse effects, through a trend for greater risk of a composite outcome of hospitalization for HF or cardiovascular death was seen in patients with transferrin saturation (Tsat ≥ 24% (3-9). However, myocardial iron was not measured in these trials.
This study also highlighted the problem of using ferritin levels to gauge adequacy of the iron stores. As we can see from the findings of this study, ferritin is a good gauge for iron stores within the spleen but is not a good measure of myocardial labile iron pool.
In summary, while iron infusion is a convenient way to quickly address severe iron deficiency and up until now did not appear to have any longer term adverse effects, accumulation of iron within the myocardium may have adverse effects similar to iron-overload cardiomyopathy.
References:
- Mayra Vera-Aviles, Syeeda Nashitha Kabir, Akshay Shah, Paolo Polzella, Dillon Yee Lim, Poppy Buckley, Charlotte Ball, Dorine Swinkels, Hanke Matlung, Colin Blans, Philip Holdship, Jeremy Nugent, Edward Anderson, Michael Desborough, Stefan Piechnik, Vanessa Ferreira, Samira Lakhal-Littleton, Intravenous iron therapy results in rapid and sustained rise in myocardial iron content through a novel pathway, European Heart Journal, 2024;, ehae359
- Olson LJ, Edwards WD, Holmes DR Jr, Miller FA Jr, Nordstrom LA, Baldus WP. Endomyocardial biopsy in hemochromatosis: clinicopathologic correlates in six cases. J Am Coll Cardiol 1989;13:116–20
- Moon JC, Messroghli DR, Kellman P, Piechnik SK, Robson MD, Ugander M, et al. Myocardial T1 mapping and extracellular volume quantification: a Society for Cardiovascular Magnetic Resonance (SCMR) and CMR Working Group of the European Society of Cardiology consensus statement. J Cardiovasc Magn Reson 2013;15:92
- Lakhal-Littleton S, Cleland JG. Iron deficiency and supplementation in heart failure. Nat Rev Cardiol 2024
- Ponikowski P, van Veldhuisen DJ, Comin-Colet J, Ertl G, Komajda M, Mareev V, et al. Beneficial effects of long-term intravenous iron therapy with ferric carboxymaltose in patients with symptomatic heart failure and iron deficiency. Eur Heart J 2015;36:657–68.
- Kalra PR, Cleland JGF, Petrie MC, Thomson EA, Kalra PA, Squire IB, et al. Intravenous ferric derisomaltose in patients with heart failure and iron deficiency in the UK (IRONMAN): an investigator-initiated, prospective, randomised, open-label, blinded-endpoint trial. Lancet 2022;400:2199–209
- Mentz RJ, Garg J, Rockhold FW, Butler J, De Pasquale CG, Ezekowitz JA, et al. Ferric carboxymaltose in heart failure with iron deficiency. N Engl J Med 2023;389:975–86
- Cleland JGF. Defining iron deficiency in patients with heart failure. Nat Rev Cardiol 2024;21:1–2
- Bailie GR, Larkina M, Goodkin DA, Li Y, Pisoni RL, Bieber B, et al. Data from the dialysis outcomes and practice patterns study validate an association between high intravenous iron doses and mortality. Kidney Int 2015;87:162–8